Solar 101 + Electricity Essentials: Exploring Solar System Ratings + Key Factors for Performance
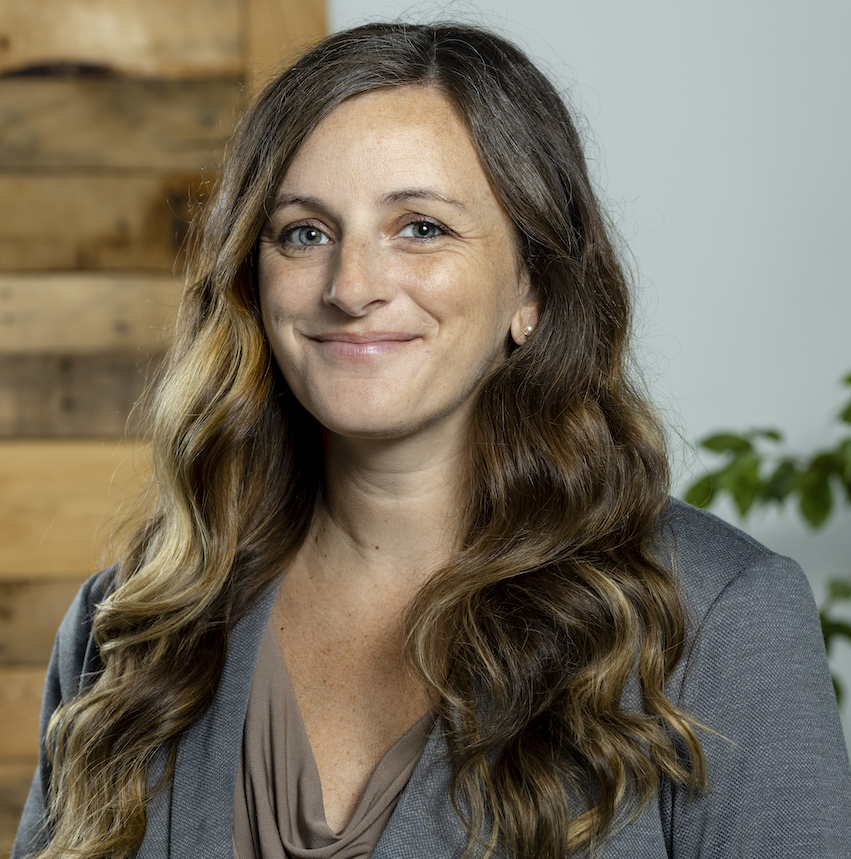
Oct 21, 2021
by Tara McDermott
So you’re looking to go solar – they’re telling you that you need a 10kW system but your neighbor with the same house as your needs a 12kW system. Then your friend one town over has the same electric bill as you, but they need a smaller system. Confusing! Why?
Or, maybe you already have solar. You bought a 7.6 kW system, but it’s the middle of summer, it’s a clear sunny day and your system is showing a current production of 5.6 kW. What does it all mean?
First, let’s explore some basic definitions when it comes to solar and electricity 101 to help you understand how your system was designed to work and produce the appropriate amount of energy you need. The most common concept that gets mixed up is kW vs kWh – or you may think of them as Energy vs Power, so we’ll start here.
Section 1: Electricity Essentials
Energy and Power
Power is a measure of electrical production, but specifically, power is a measure of production at a snapshot in time. Although it seems to get confused a lot as an amount of energy production, power is really just a rate of energy production. Most people in the solar industry refer to power as watts or kilowatts, e.g. a 5 MW solar system. Power is measured in kW (or mW.)
Energy is the amount of power produced over a length of time. This is a really important one to remember when you’re researching solar products. You want to find out how much energy a solar product will actually produce not just the power that it can produce at a moment in time. Energy is measured in kilowatt-hours (kWh).
Here’s our next equation:
Power x Time = Energy
Okay, time for an analogy. Imagine that you’re driving a car. Power is the speed you’re going at the moment you look down at your speedometer. Energy is the total distance you’ve traveled. See? It’s really simple after all.
In addition to energy & power, we also want to make sure we cover some other helpful electricity basics here. This may only be a refresher course for some of you, but regardless of how much you know, you should feel more comfortable with several key electrical and solar power terms after this post. This should allow you to better understand solar power and feel more confident for future decisions.
Electricity 101
Okay, so electricity has three key terms: amperes (or amps), volts, and watts. Current (amperes) is a measure of the flow of electrons. Volts are a measure of electric potential. Watts measure the amount of power supplied by a given amount of current at a certain voltage. In case there’s a random pop quiz in the office, the math looks like this: Amperes (current) * Volts (potential) = Watts (power output)
Technically speaking, electricity is a current (flow) of charged particles called electrons, but instead, let’s think of it as water moving through a hose. The electric current, or amperage, is like the size (diameter) of the hose. The voltage is like the amount of pressure behind it. The greater the size and pressure are, the greater the rate of water flowing from the hose. So, the more the amps and volts in a wire, the more watts will come out of it.
Irradiance and Insolation: Measuring the Sun
Honestly, we’re almost through the definitions, but believe us; understanding these definitions will make it a lot easier to discuss solar power after our review. Next up are irradiance and insolation. Irradiance is the amount of sunlight available at a moment in time.
Insolation is a measure of the irradiance over a period of time. Here’s another equation to remember for your next appearance on a trivia game show:
Irradiance x Time = Insolation
Irradiance is the most important factor in solar power production. The higher the irradiance, the more sunlight hits the solar cells. That means more of those little electrons get boosted out of the semi-conductor material to flow through the circuitry. The more electrons flow, the more electrical power gets produced. So there you have it: lots of sun means lots of electricity.
Solar Efficiency: Converting the Sun to Electricity
At last, we’ve come to our final term of the day. Solar efficiency refers to the amount of sunlight that can be converted into usable electricity. Solar photovoltaic efficiency is often discussed as solar cell efficiency or solar panel efficiency. Why is this distinction important? A lot of times, people may quote you amazing cell efficiency numbers. That sounds great, but what you really want to know is the solar panel efficiency. The solar cell efficiency number is only for the amount of light that a single solar cell can convert to electricity. But solar panels are made up of multiple cells, and the spacing between cells among other things reduces the overall efficiency. That means that the efficiency of a solar panel will typically be lower than that of a solar cell.
So the panel’s efficiency will be a better predictor for the actual electricity that the system can generate. It really doesn’t matter if a company’s solar cell has a 43% efficiency if their panel’s efficiency is 6%. (We’re exaggerating here, but you get the point. The top solar panel efficiencies now-a-days are around 22%, by-the-way). Therefore, you’ll want to pay attention to the solar panel efficiency number when shopping around.
You may be hearing a lot of promises about how much output or savings you’ll get with different solar technologies. To help you get a clear picture, we’re going to go into a little more depth about the factors that influence solar energy production. Understanding these factors will help you to make accurate predictions for cost-savings and smart decisions on choosing a product. With that in mind, here are several factors and how they influence solar energy production.
Section 2: Key Factors for Solar Performance Factors for Solar Performance
It’s a fact that the weather changes every day, and the earth’s position towards the sun changes throughout the year. That means that a solar panel at a fixed location may produce 50 W at noon in December and 100 W at noon in June. Similarly, the same panel may produce 120 kWh per year in one geographic location and only 95 kWh in another. In both situations, the production difference is largely because of differing amounts of sunlight. But other external factors like temperature, shading, weather, and soiling also affect the total energy a system can produce.
Solar Efficiency: The Central System Factor for Performance
Before getting into the external stuff, let’s take a moment to reinforce the importance of solar panel efficiency. This is pretty straightforward: The more efficient a solar panel is, the more electricity it can create from sunlight. A solar panel with 18% efficiency converts more light to electricity than a solar panel with 12% efficiency. Nothing tricky about this solar fact. Consequently, high efficiency solar panels often offer the best economics because they will:
- Generate more electricity with fewer panels
- Require less space than lower efficiency solar panels
- Offer more long-term savings
When you’re looking for solar panels, you can expect to be quoted solar efficiencies ranging from 6% to 20%. So be sure that you compare solar panel efficiency rates and not solar cell efficiency rates. By the way, you might want to ask about efficiency loss from initial break-in. High quality solar panels will maintain their rated efficiency, but lesser quality solar panels may lose up to 3% of efficiency during their initial exposure to sunlight. That’s almost as bad as all the value you lose in driving a new car off the lot. But in this case, this loss is totally avoidable.
5 Critical External Factors Influencing System Performance
1. Irradiance
We’ve talked about irradiance before, but it bears repeating since it’s not exactly an everyday word. You just don’t hear people say, “Hi. How are you? Man, that irradiance outside is killer today.” Nope. Not normal speech for most of us. Essentially, irradiance is a measure of the amount of sunlight falling on a given surface. The higher the irradiance on a solar cell, the more energy a cell will produce. More sunlight = more electricity. If only sunlight were constant. The fact is that irradiance varies throughout the day. The angle of the sun, passing clouds, hazy weather, and air pollution can affect irradiance levels. However, the total energy received by the system from the sun remains relatively constant from year to year. Typically, energy from the sun only varies between 5-10% of the average in a given year. Consequently, quality solar energy output projections can be made based off of past years (Usually the data comes from national weather databases, but we’ll talk more about that later.)
2. Temperature
Here’s an interesting fact about solar power: the warmer solar cells get, the less efficient they are. This may be surprising, but think about walking through air versus walking through water in a pool. You move pretty quickly with only air around you. You’re not quite as quick walking through water because of the resistance. Same goes for those little electrons. The hotter the cell material is, the more resistance there is and the slower the electrons can move through it. This means that production goes down because not as many electrons can get through the circuitry in the same amount of time as before. This is another situation where quality matters. High quality panels are designed to maintain performance levels in extreme heat. Lower quality panels lose efficiency and produce less energy. What a bummer. We can’t imagine making a huge investment only to watch it underperform on a July afternoon when it should be creating lots of free electricity.
3. Shading
This is a no-brainer: shaded solar panels produce less electricity. One thing to consider is that shading varies seasonally. As the angle of the sun changes through the year, trees and other barriers may become shading issues in different seasons. It all depends on the size, height, and proximity of surrounding barriers. Properly designed solar systems minimize or eliminate shading. Under some circumstances, it is not possible to avoid all shading, so proper design will minimize it during peak midday production periods.
You’ll want to pay attention to the effects of adding future roof-top structures (like putting up an enormous Santa in a sleigh with eight reindeer around x-mas time). You’ll also want to minimize the effects of shading from nearby tree growth.
4. Soiling
This is another no-brainer. Dirty solar panels produce less electricity. The term “soiling” sounds fancier than it is. All it refers to is dust, dirt, and other debris settling on the surface of the solar panels. This blocks sunlight from reaching the solar cells and reduces solar system performance. In areas with frequent rain, soiling is not usually significant. Areas that experience long periods of dry weather, such as California and the desert Southwest, experience more soiling during the summer. Rapid soiling can also occur on systems located near construction sites and other places that produce dust. Cleaning the system may be undertaken to keep things looking nice, but it shouldn’t be necessary to maintain the expected energy cost-savings from a properly-designed system.
5. Snow
Snow blocks production of solar energy until plowed or melted. (Seriously, we’re just being thorough. We know you know this.) Because snow limits energy production, the effects of snow should be incorporated into any energy estimates. In general, assumptions for performance degradation may range from a minimum of 8% performance degradation (for early spring and late fall months) to a maximum of 30% (for mid-winter months) for systems in regions that experience significant snowfall.
As you can see, there are a lot of factors that influence solar production. That can make it hard to tell if your panels are living up to their solar ratings. However, a few handy formulas can make it easy to compare real world power production and rated production. Let’s start off with two essential benchmarks for your comparisons: energy production and peak power production.
Section 3: Real World Expectations for Your Solar System
Understanding System Performance Terms
Energy Production
These terms should be looking more familiar at this point if you weren’t already aware of them. In our first section, we talked about energy and power. Expressed in kilowatt-hours (kWh), energy production is the first and most important benchmark of system performance. The predicted energy production of a system is based on local, historical weather patterns. Because energy is measured over a period of time, energy production does not require many adjustments for an accurate comparison between forecast production and actual production. The differences between the two are usually because of variations in sunlight, i.e. there was more or less sunlight than the forecast assumed. Whenever possible, we highly encourage you to use energy production as your main benchmark for comparison because it is actually what your system has produced.
Peak Power Production
Power production at a specific moment in time (instantaneous power production) is the second performance benchmark. It is commonly discussed as kilowatts (kW), and this is where the fun begins. This measure is not as reliable as the energy benchmark. However, it can be evaluated quickly by normalizing performance data. If normalization is a new term for you, just think of it as a way to level the playing field. Essentially, normalization is a way to adjust values measured under actual conditions to compare them directly to power rating values under standard test conditions (STC) or other ratings. For example, how a solar system is performing relative to its AC rating at specific irradiance and temperature conditions can be estimated with very good accuracy. In both situations, the production difference is largely because of differing amounts of sunlight. But other external factors like temperature, shading, weather, and soiling also affect the total energy a system can produce. ©201
Normalizing Performance Data
Normalize the irradiance. Example: A system is producing 80 kWac at 900 W/m², but the AC rating conditions require 1000 W/m². Power normalized to irradiance is: 80 kWac * (1000/900) = 88.9 kWac.
Short and sweet for that one, right?
- Normalize the temperature.
All right, we admit it. This one is a little more complex, but it’s the last of the math for now. For crystalline solar panels, the temperature factor varies from -0.35%/ °C to -0.55%/ °C. A typical value is -0.45%/ °C.
Example: The ambient temperature measured at the system site referenced above is 35 °C. Rating conditions (PTC) require 20 °C. Power normalized to temperature (and irradiance) is: 88.9kW * (100% + (20 °C – 35 °C)*(-0.45%/ °C)) = 94.9 kWac.
An additional step can be taken for wind speed and air mass, but you get the idea. There are different equations that will allow you to determine if a solar system is performing up to its rated performance or not.
Setting Performance Expectations for Solar System Performance
Energy
As we’ve discussed before, a solar system will produce different amounts of energy each year depending on the available sunlight. Some years, a solar system will produce more than predictions, and some years it will produce less. Energy production from year to year can vary by as much as 20%. But don’t worry. Generally, it will remain fairly consistent. When you’re working with a quality solar provider, you can expect that the average annual energy production will be within one or two percent of the performance simulation.
Power
Since most of the industry talks about watts and kilowatts, we’re spending quite a bit of time explaining power. These terms are important, but you are probably realizing that energy production (kilowatt-hours) is much easier to understand. Power can range widely because solar system power output is highly dependent on the local conditions, time of day, season of the year, geographic location, and so forth.
For example, many people expect that if their solar system capacity is rated at 100 kWac, then the system will produce 100 kW on any sunny day. But it won’t. A 100 kWac system is not expected to produce 100 kW on average during any month of the year in any geographic location. It is perfectly normal for a 100 kWac rated system to produce an average of 70 kW at noon in June. As we mentioned in the solar ratings section of this blog, standardized conditions usually occur for a few minutes of just a few days in any year.
And geography plays a big role because of the different solar resources available around the globe. For example, consider a 100 kW system located in New York and California. Power production on an average day in California is greater than that of an average day in New York in every month of the year. So clearly geography plays in major role in solar production.
Section 4: Understanding Solar System Ratings
Why Do Solar Ratings Matter?
In our Electricity Essentials solar section, we laid down some groundwork to help you understand key terms. Now, we’re going to tackle another topic that can be confusing: solar ratings. Solar power ratings allow you to
make direct comparisons between solar panels of different sizes from different manufacturers. Sounds good, right? Well, there’s more than a little scientific and industry jargon to wade through. Don’t worry. We’re going to simplify it for you, and when we’re done, you’ll feel more confident in making comparisons and accurately predicting energy output and savings for different solar products.
Understanding Solar System Ratings
What Is a Solar Rating?
Solar photovoltaic (PV) panels are classified (or rated) by the power they produce under specific conditions. The most common ratings used in the industry are peak/STC, PTC, CEC-AC, and AC. Take a deep breath. They’re just acronyms. Let’s start with the first one.
Peak/STC Rating
Every solar panel has a published power rating. This is its rated power under Standard Test Conditions (STC). If you add up the rated power for all of the panels, then you get the peak rating of a solar system. The STC rating is always the highest rating. This is because it rates solar panels in terms of the instantaneous power that they produce under a set of ideal conditions. But when do ideal conditions ever exist in anything in this world? The reality is that solar panels will produce different amounts of power throughout a year. That’s why the other ratings were created- to give people a more accurate picture.
Standard test conditions are defined as follows:
- 1000 W/m² solar insolation
- 25 °C solar cell temperature (which assumes roughly 0 °C ambient temperature)
- Absolute air mass of 1.5
Let’s pause for a moment to break this down a little. We talked about insolation previously. That’s just a term for sunlight. The temperature metric takes into account increases or decreases in production due to heat. The last metric accounts for effects from atmospheric pressure. All this does is to standardize the way panels are evaluated. You won’t need to know the math behind this.
Two last things about peak ratings:
- These ratings are measured in terms of direct current (DC).
- They require a high level of solar radiation at a very low temperature to produce the rated amount of power. (That means their production values are very rare, occurring on only a few mild, clear spring or summer days.)
PTC Rating
Here’s today’s fast-fact that you will otherwise never need to know about: The PVUSA Test Conditions (PTC) DC rating came out of work by the Photovoltaics for Utility Scale Application (PVUSA) team in the late 1980s and early 1990s. PTC rating conditions are defined to be:
- 1000 W/m² solar insolation
- 20 °C ambient temperature at 10 meters above ground level
- 1 m/s wind speed
- Absolute air mass of 1.5
So, you can see the similarities with the STC ratings. It’s a little more realistic, right? It takes into account influences from the wind (because solar panels are going to experience some amount of wind being outside, of course), and the rating standardizes against a slightly lower temperature. As you can tell by the name, this is also rated in terms of direct current. PTC-DC ratings are published by the California Energy Commission (CEC) if you need to go find them.
CEC-AC Rating
The CEC-AC system rating is used to determine the eligibility of a solar system for the California Solar Initiative (CSI) Program. But even if you’re not in California, this rating is closer still to real world production. Therefore, it can still be helpful in your comparison shopping, and that’s why we’re telling you about it. This rating uses the PTC-DC rating of the solar panels and multiplies it by the number of solar panels and the inefficiency introduced by the DC to AC inverter. The inverter efficiency is usually around 95% in case you’re curious. Here’s the basic equation:
PTC Rating x Number of Panels x Inverter Efficiency = CECAC Rating
As you can tell, we’re getting closer and closer to actual production values.
AC System Rating
The AC system rating is based on actual simulations of the solar system, and it attempts to account for all possible inefficiencies. In addition to the inverter inefficiency, the AC system rating includes inefficiencies from shade, soiling, wiring energy losses, and trans former energy losses. The AC system rating is usually 80% of the peak rating, but it is still based on the PTC rating conditions. Obviously, since the AC system rating takes into account multiple inefficiencies, it is the most accurate rating. A solar system should produce power at its AC rating at noon on a very clear, cool day.
The Key Rating Takeaway
So why wouldn’t you just go with the AC system rating for all your evaluations? Well, sometimes it’ll just be easier to get the STC ratings from 3 different solar providers as you do your homework. What’s important is that you’re making an apples-to-apples comparison by using the same ratings. That’s the big take-away from this post. So if you’re getting STC ratings from one solar provider, you’ll want to get STC ratings for all other solar products. You don’t want to be comparing a CEC-AC rating against an STC rating for 2 different solar panels. It just wouldn’t make any sense, and it could lead to a poor decision like choosing the STC rated panel because it seems high.
In Summary
There you have it. That’s the basic overview of solar electricity 101, key performance factors and a look at ratings. We hope that it’s cleared up any confusion might have about your system or provided help while you’re researching the right type and size system for your home. As we now know, there are a number of factors that influence how much electricity a solar system can produce; mainly sunlight and solar efficiency.
Content developed by SunPower. ©2015 SunPower Corporation. All Rights Reserved.
Save up to 30% on day one and say goodbye to rising electric bills
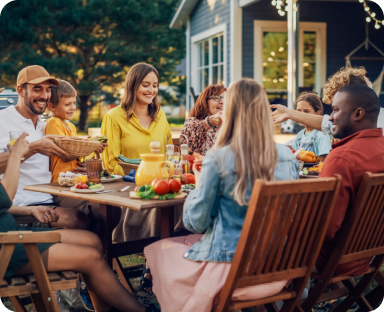
Save up to 30% on day one and say goodbye to rising electric bills
About the Author
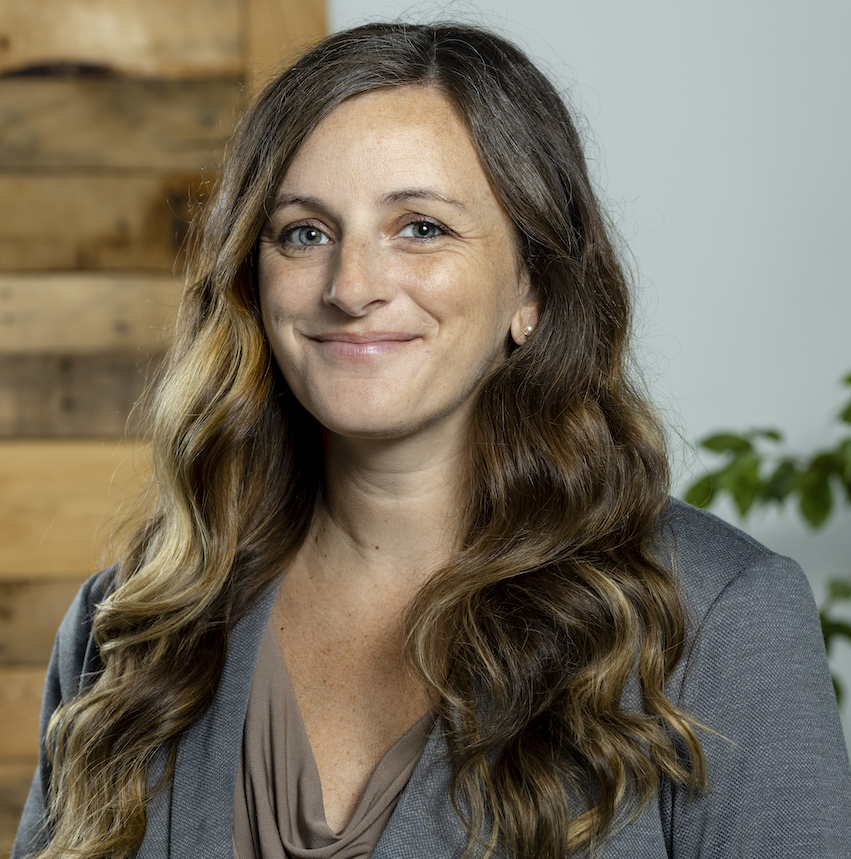
Tara represents EmPower Solar as the Chair of the Long Island Solar & Storage Alliance where she is the government liaison for policy issues related to solar and energy storage. In 2018, Tara was named one of the Top 50 Women in Business by the Long Island Business News. She was also inducted into the Social Justice Distinguished Scholar Academy for her work in environmental justice issues in collaboration with Farmingdale State College.